Secondary cratering on Earth: The Wyoming impact crater field: More than three question marks. – Comment on the Kenkmann et al. article (GSA Bulletin).
Kord Ernstson, Hans-Peter Matheisl, Jens Poßekel and Michael A. Rappenglück
April 2022
A short version has been accepted as an abstract paper (for poster presentation) for the 85th Annual Meeting of the Meteoritical Society 2022 in Glasgow, Scotland (6079.pdf, LPI Contrib. No. 2695):
The Wyoming Impact Crater Field: Secondary Cratering vs. Primary Cratering.
Abstract, table of contents and the full article follow here directly for reading.
Abstract. – Secondary craters in impacts on moon, planets and their moons are a well known phenomenon, which has been investigated many times. In the article commented by us here, the authors report on a crater strewn field in the American state of Wyoming, which is interpreted as a field of secondary craters of a so far unknown larger primary impact structure and as a first on Earth. We compare the Wyoming crater strewn field with the Chiemgau impact crater strewn field in SE Germany and find that both have nearly identical characteristics of virtually all relevant features, in terms of geometries and petrography. We conclude that the alleged Wyoming secondary crater field is a fiction and the craters attributable to a primary impact. The alleged evidence is very poor to easily refuted. A primary crater does not exist to this day. The negative free-air gravity anomaly referred to, but not even shown, is invalid for this purpose. The Bouguer gravity map shows no indication of a possible large impact structure. Also unsuitable is the use of asymmetries with elongations of assumed secondary craters with a very questionable corridor intersection for the ejecta. Of 31 craters surveyed as proven, 15 are circular (eccentricity 1) and more than half (19) have an eccentricity ≤1.2. Circular and elongated craters are intermixed. The evaluated crater axes may just as well originate in a multiple primary impact. Elongated craters may also result from doublets of overlapping craters that are no longer fresh, as described by the authors themselves. In their paper, the authors do not show a Digital Terrain Model with contour lines for any of the surveyed craters, but only aerial photos blurred by vegetation. A verification of the crater measurements with the deduced eccentricities and strike directions is impossible. Not a single topographic profile over even a single crater in the strewn field is shown, either from DTM data or from an optical leveling, which could have been accomplished in an instant given the relatively small craters. Grave is the misconception that such a large crater field of 90 km length with four separate clusters is not possible according to 20 years old model calculations. A primary impact with multiple projectiles could perhaps be conceivable under rare circumstances, which are described by the authors as not relevant. The alleged impossibility of such a large primary strewn field with referring to the known small impact fields of Morasko, Odessa, Wabar, Henbury, Sikhote Alin, Kaalijärv, and Macha is contradicted by the three larger impact strewn fields of Campo del Cielo, Bajada del Diablo (very likely), and Chiemgau, which are best described in the literature but are not mentioned by Kenkmann et al. with a single word. The comparison of the Wyoming strewn field with the Chiemgau impact crater strewn field of about the same size here in the commentary article proves the scientifically clearly much greater significance of the Chiemgau impact, which must be considered as currently the largest and most significant Holocene impact despite the rejection and ignoring in some parts of the so-called impact community.
GSA Bulletin Kenkmann et al. article. (Kenkmann, T., Müller, L., Fraser, A., Cook, D., Sundell, K., and Rae, A.S.P)
Article Editors: Rob Strachan, W.U. Reimold
_____________________________________
Key Words: secondary impact cratering, Wyoming, Chiemgau impact, impact crater strewn fields, Bajada del Diablo, Campo del Cielo

1 Introduction
In February 2022, authors Thomas Kenkmann, Louis Müller, Allan Fraser, Doug Cook, Kent Sundell, and Auriol S.P. Rae published an article in the GSA Bulletin claiming that a secondary crater field of a major impact structure has been detected for the first time in the state of Wyoming in the United States, as has long been known from the Moon, other planets, and their moons. 31 craters are confirmed by shock effects, and more than 60 are considered possible craters. Two findings are highlighted as evidence: the axial directions of elongated craters within four separately occurring clusters of craters. The elongated axis directions span four acute-angled corridors that overlap at a distance of roughly 200 km, where they are thought to mark the presumed primary crater from which projectiles were launched along the corridors to create the secondary craters. The landing of these projectiles after ballistic trajectory are then supposed to have produced the elliptical to ovoid asymmetric shapes of the secondary craters, although more than half of the measured craters are circular structures. In the intersection region of the four corridors, according to the authors, there should rightly exist a significant negative gravity anomaly of a hypothesized primary impact crater measuring perhaps 50 km. Such a large anomaly is proposed with the fundamental shortcoming that the completely unsuitable map of free-air anomalies is used in place of the correct Bouguer anomalies. Third, it is argued that such a large Wyoming strewn field, measuring close to 90 km, could never be attributed to a primary impact according to theoretical modeling and considerations.
In our commentary on the Kenkmann et al. article, we discuss not only these methodologically serious shortcomings of the paper, which culminate in the fact that such a large primary impact event with a comparably large crater strewn field has been established long ago and for 15 years. This Holocene event of the Chiemgau impact in SE Germany can, according to the extensively published literature (Schüssler et al. 2005; Rappenglück et al. 2009; Ernstson et al. 2010, 2012; B. Rappenglück et al. 2010; Liritzis et al. 2010; Hiltl et al. 2011; Isaenko et al. 2012; B. Rappenglück et al. 2012; Shumilova et al. 2012; Rappenglück et al. 2013; Bauer et al. 2013; Neumair and Ernstson 2013; Ernstson et al. 2013; Rappenglück et al. 2014; Ernstson et al. 2014; Ernstson 2012, 2016; Ernstson and Poßekel 2017; Rappenglück et al. 2017; Shumilova et al. 2018; Poßekel and Ernstson 2019; Bauer et al. 2019; B. Rappenglück et al. 2019; Bauer et al. 2020; Ernstson et al. 2020a; B. Rappenglück et al. 2020; Poßekel and Ernstson 2020; Ernstson and Poßekel 2020; Ernstson et al. 2020b; Rappenglück et al. 2021), be described as probably the most important terrestrial impact crater strewn field at present, leaving the Wyoming strewn field now described far behind in scientific importance. This great Chiemgau impact is not mentioned with a word in the Kenkmann et al. article.
The core of our commentary article makes up for this and contrasts Wyoming and Chiemgau strewn fields in greater detail.
2 The Chiemgau (SE Germany) impact crater strewn field
The Chiemgau impact strewn field discovered in the early new millennium and dated to the Bronze Age/Celtic era comprises more than 100 documented (and more than 100 additional suspected) rimmed craters scattered in a region of about 60 km length and ca. 30 km width in the very South-East of Germany (Fig. 1). The crater diameters range between a few meters and a few hundred meters, among them Lake Tüttensee with a rim-to-rim diameter of about 600 m and an extensive ejecta blanket. SONAR echosounder measurements establish a doublet crater with a ring wall at the bottom of Lake Chiemsee measuring about 800 m x 400 m (Ernstson 2012) Impact tsunami deposits are observed around Lake Chiemsee (Ernstson 2016). Since a few years, confirmed by gravimetry and ground penetrating radar measurements and DTM analyses, the 1.3 km-diameter Eglsee crater has joined as the largest crater in the strewn field so far, showing astonishing morphological similarity to the Barringer crater (Ernstson 2017, Ernstson and Poßekel 2020).
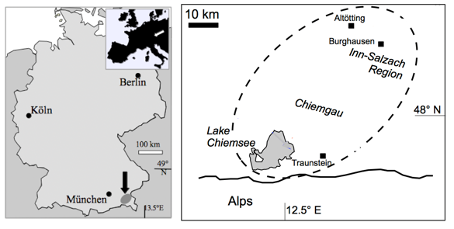
Fig. 1. Location map for the Chiemgau meteorite impact strewn field.
Geologically, the craters occur in Pleistocene moraine and fluvio-glacial sediments. The craters and surrounding areas are featuring heavy deformations of the Quaternary cobbles and boulders, abundant fused rock material such as impact melt rocks and various glasses, strong shock metamorphism (planar deformation features [PDFs] in quartz and feldspar, diaplectic glass from quartz, feldspar and muscovite, ballen structures in silica, toasted quartz), geophysical (gravity, geomagnetic, seismic, ground penetrating radar) anomalies (Ernstson et al. 2010; Neumair and Ernstson 2011, Rappenglück et al. 2017) and widespread impact-induced rock liquefaction features (Ernstson et al. 2011, Ernstson and Neumair 2011, Ernstson and Poßekel 2017). Impact ejecta deposits in a catastrophic mixture contain polymictic breccias, shocked rocks, melt rocks, and artifacts from Neolithic and Bronze Age/Iron Age people (Ernstson et al. 2010, Rappenglück et al. 2017, and references therein). The impact is substantiated by the abundant occurrence of metallic, glass and carbonaceous spherules, accretionary lapilli and microtektites (Ernstson et al. 2012, 2014). Strange, probably meteoritic matter in the form of iron silicides like gupeiite, xifengite, hapkeite, naquite and linzhite, various carbides like, e.g., moissanite SiC and khamrabaevite (Ti,V,Fe)C, and calcium-aluminum-rich inclusions (CAI), minerals krotite and dicalcium dialuminate (Hiltl et al. 2011; Rappenglück et al. 2014, Rappenglück 2022) add to the finds. Carbonaceous spherules contain fullerene-like structures and nanodiamonds that point to an impact-related origin (Yang et al. 2008). Such spherules were found embedded in the fusion crust of cobbles from a crater as well as a possible outfall in soils widespread over Europe (Rösler et al. 2005; Hoffmann et al. 2005, 2006; Yang et al., 2008). Abundant finds of glass-like carbon fragments with pumice texture, which has been given the name chiemite, contain the carbon allotropes diamond and carbyne in a largely amorphous matrix of more than 90 % carbon (Shumilova et al. 2018, Ernstson and Shumilova 2020). A formation of a direct airburst shock transformation of the target vegetation (wood, peat) to carbon melt and vapor in the impact event is suggested.
Physical and archeological dating confines the impact event to have happened between 900 and 600 B.C. (Rappenglück et al. 2010; Liritzis et al. 2010, B. Rappenglück et al. 2020, 2021). The impactor is suggested to have been a roughly 1,000 m sized low-density disintegrated, loosely bound asteroid or a disintegrated comet in order to account for the extensive strewn field (Ernstson et al. 2010, Rappenglück et al. 2017). Lots of intriguing observations in the strewn field suggest a giant air burst or a cloud of airbursts that have accompanied the impact event (Rappenglück et al. 2020, Ernstson 2018).
3 The Wyoming and Chiemgau impact strewn fields: a comparison
3.1 Characteristics, size and shape of both strewn fields
In Fig. 2 the strewn fields of the Wyoming and Chiemgau impacts, which serve for comparison, are placed side by side, and it does not require too much imagination to recognize a basic relationship. A length of 90 km and a width of 40 km for the Wyoming strewn field are compared with a length of 60 km and a width of about 30 km for the Chiemgau strewn field. The former counts 90 confirmed (with shock effects) and possible impact structures. In the Chiemgau strewn field, after originally 80 defined craters, meanwhile far more than 100 craters are counted, whereby an increase up to about 200 and more seems realistic, which is mainly due to the fact that with the DTM also in forest and swamp areas crater structures, partly in clusters, are increasingly discovered.
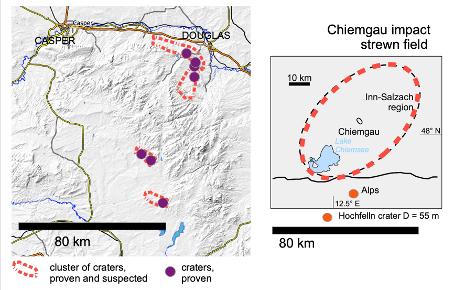
Fig. 2. Location maps for the Wyoming and the Chiemgau impact strewn fields. Left: OpenTopoMap and Kenkmann et al. (2022); right: modified from M.A. Rappenglück et al. (2017).
Increasingly, with the help of the DTM, craters are also recognized in the first foothills of the Alps, together with reports from amateur researchers about accompanying debris fields. A classic example is the 55 m diameter Hochfelln crater marked in Fig. 2 (Fig. 10).
3.2 Crater morphologies and structures
The crater morphologies and structures of the Wyoming craters read as follows in the Kenkmann et al. article. The letters index the following sections with their respective images, captions and text for the Chiemgau counterparts.
— Diameters between 10 m and almost 70 m. (A)
— Circular, irregular-shaped, and ellipsoidal impact structures. The freshest craters have distinct elliptical to ovoid crater shapes. (B)
— Fresh craters contain steep crater walls and raised rims (C),
— with overturned ejecta flaps, (D)
— with remains of the proximal ejecta blankets (E), partly asymmetrical (E)
— Irregular crater clusters and crater chains, where craters partly overlap. (F)
— Crater structures, circular in outline, show internal ring features instead of a central morphological depression (G)
— Occasionally linear ejecta (herringbone features) (H)
— Estimates of the apparent d/D ratios are in the order of 0.1 and less and are determined in their present state (J).
We find that in the Chiemgau impact crater strewn field practically identical forms occur, not as random single phenomena, but on a regular basis, which can be substantiated in the following with a selection of characteristic examples only to a limited extent.
The DTM images result from a data processing in different representations as contour maps, shaded relief maps and 3D surface maps.
A: Diameters (rim-to-rim) – Chiemgau impact, Digital Terrain Models (DTM).
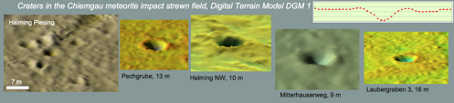
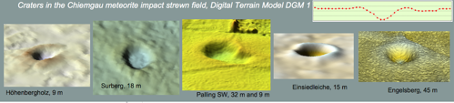
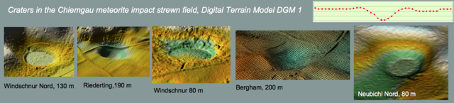
Fig. 3. Selection of impact craters in the Chiemgau strewn field. DTM surface. For the data processing, it should be noted that for part of the craters, which is also true for the following sections, terrain trend fields have been calculated and subtracted using low-pass filtering.
B: Circular, irregular-shaped, and ellipsoidal impact structures – Chiemgau impact DTM
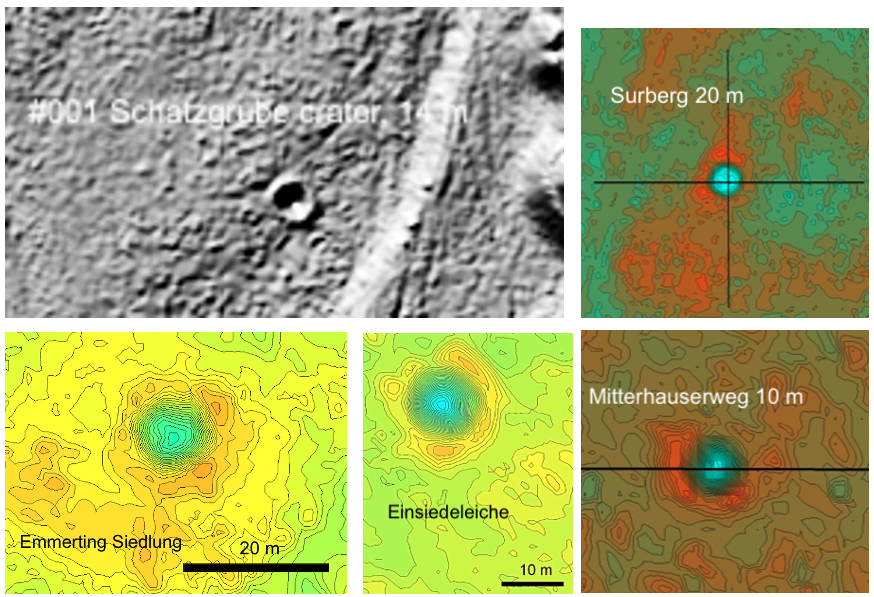
Fig. 4. Circular impact craters. The #001 Schatzgrube crater will be addressed also in the Discussion chapter. DTM shadowed relief (top left) and contour maps. Along the black lines DTM crater profiles have been taken.
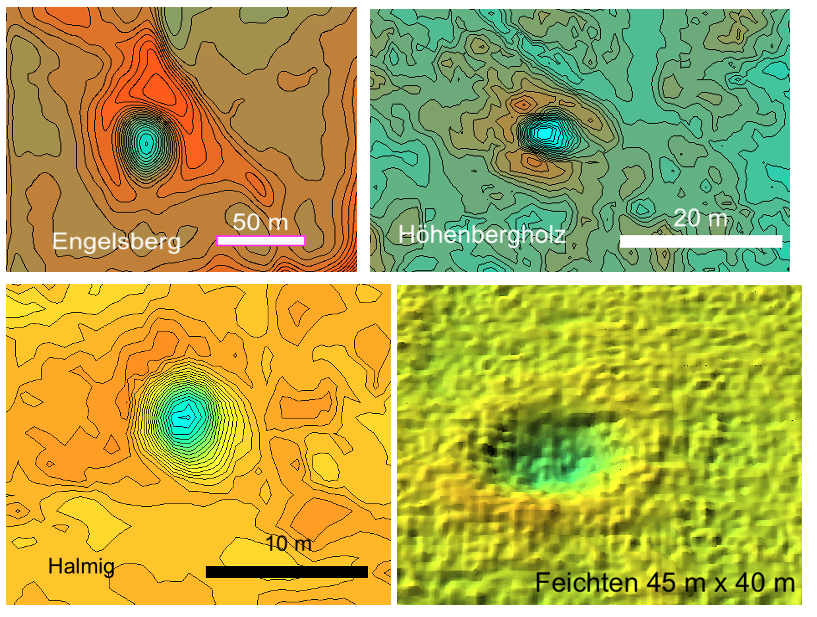
Fig. 5. Ellipsoid and ovoid impact structures. The strong pattern in the high-resolution 3D surface map of Feichten is the result of cropland management.
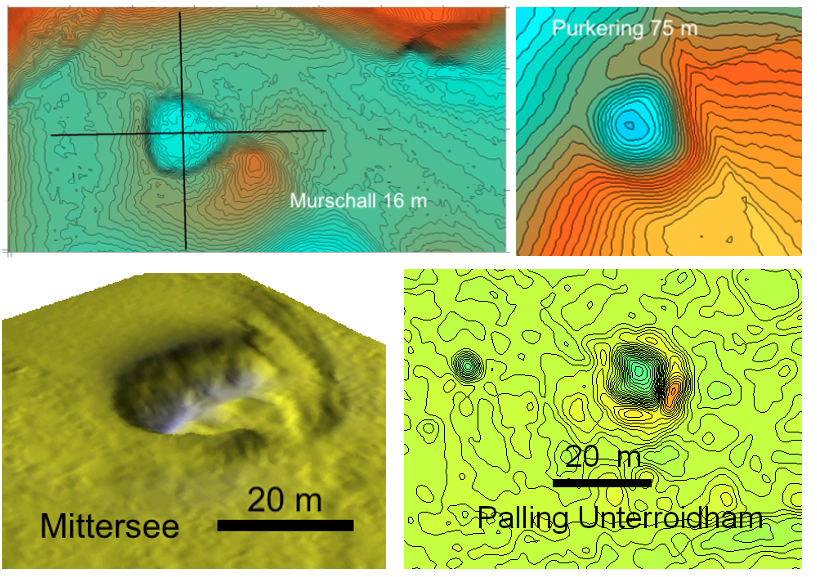
Fig. 6. Irregular-shaped impact structures. Unterroidham with a neighboring circular crater.
C: Fresh craters contain steep crater walls and raised rims, Chiemgau impact
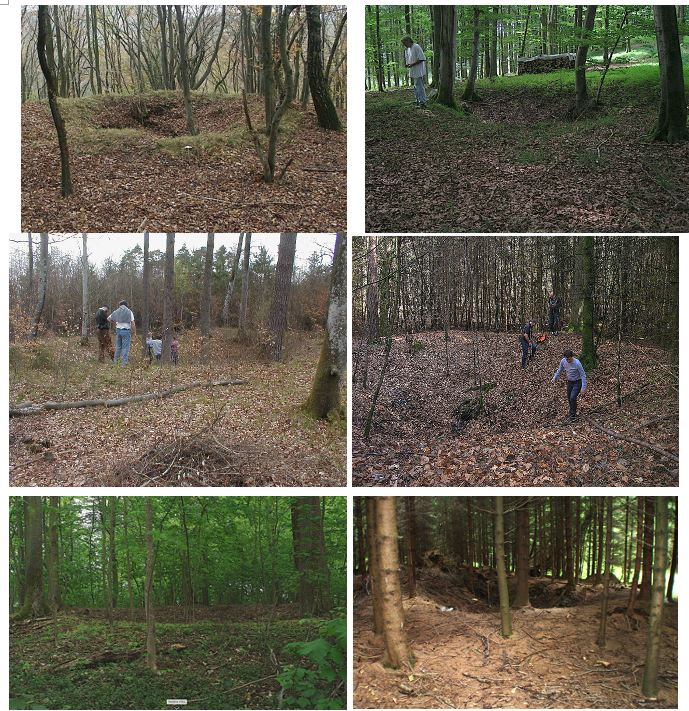
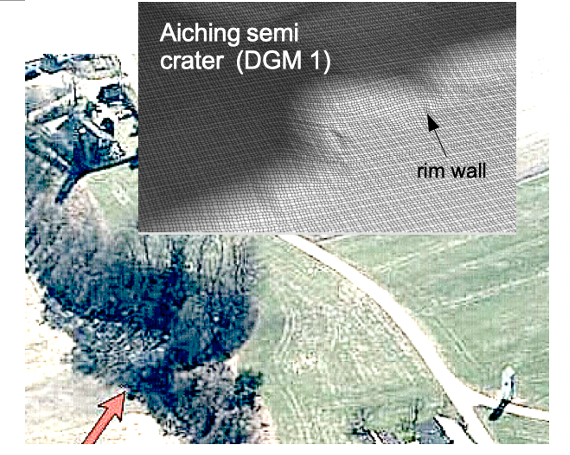
Fig. 7. Selection of Chiemgau impact craters with raised rim walls. Hohenwart, Thalham cluster (also see Fig. 16), Laubergraben, Schatzgrube, Sünching, Mauerkirchen, Aiching (Inn River valley; also see Fig. 9).
D: with overturned ejecta flaps
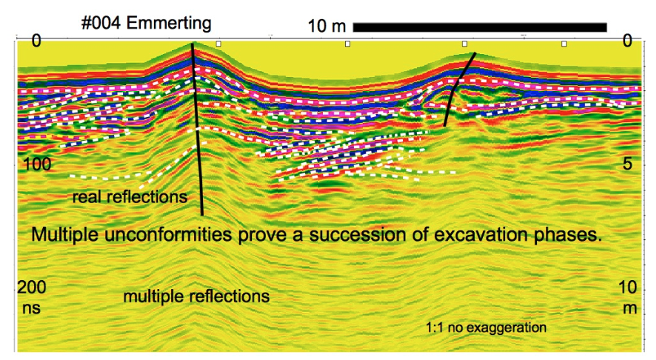
Fig. 8. Ground penetrating radar (GPR) diametral profile indicates a temporal sequence in the ejection process with evidence of overturned sedimentation. 25 MHz (plus modulated 200 MHz) antenna (RTG).
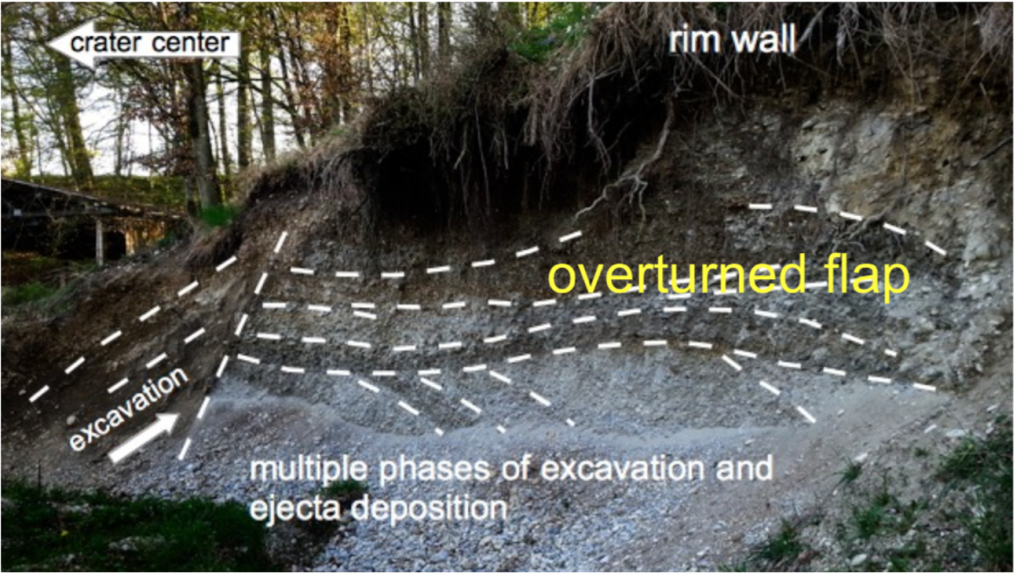
Fig. 9. Crater rim of the 60 m-diameter Aiching semi impact crater in a gravel pit. One of the very rare opportunities to observe an impact crater in a section through its crater rim. The Aiching semi crater is punched into the embankment of the Inn River.
E: remains of the proximal ejecta blankets, partly asymmetrical
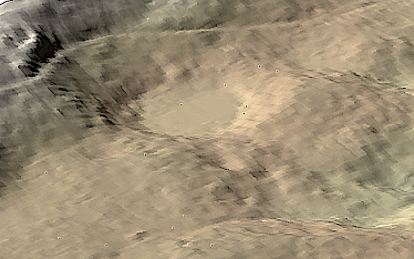
Fig. 10. Chiemgau impact, DTM surface: 55 m-diameter Hochfelln crater in the Alpine foothills with rim wall and significant ejecta blanket; mud-filled.
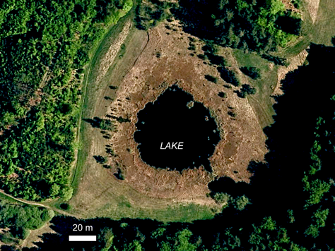
Fig. 11. Ejecta blanket of the Engering crater.
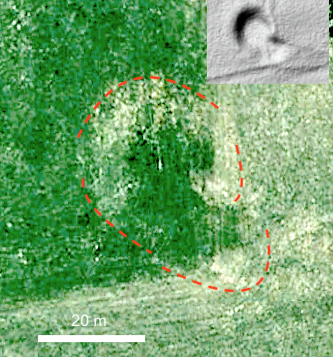
Fig. 12. Ejecta blanket of the doublet Palling SW crater. The whitish blanket is composed of coarse limestone gravel, which was excavated from depth, ejected and emplaced over the younger loess. Similar explanation holds true for Figs. 13 and 14.
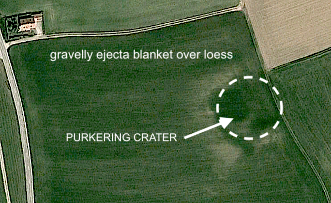
Fig. 12A . A blanket of excavated limestone gravel ejecta over target loess.
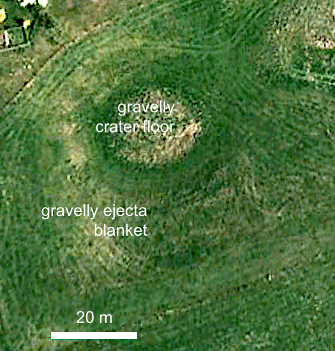
Fig. 13. General stratigraphy: Loess over gravel. Impact excavation seen in the inverted ejecta blanket. Seeon Neubichl crater.
G: Irregular crater clusters and crater chains, where craters partly overlap – Chiemgau crater strewn field
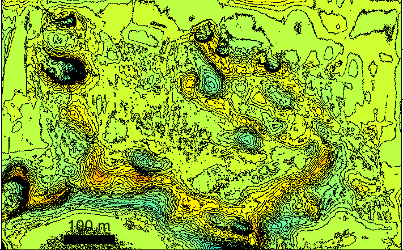
Fig. 14. Tütten-Holz crater cluster, accompanying the 600 m-diameter Lake Tüttensee crater. With respect to the Wyoming crater strewn field and the inflow trajectory derived from the elongation of assumed secondary craters, it is particularly noteworthy here that pronounced elongation in a preferred WNW – ESE direction occurs for most of the craters in the Tütten-Holz cluster, which moreover have pronounced ring walls. A special unique feature for the elongation of the Wyoming craters can therefore not be deduced. The openings in the ring wall, which can be seen in some craters, can undoubtedly be explained by gravel extraction.
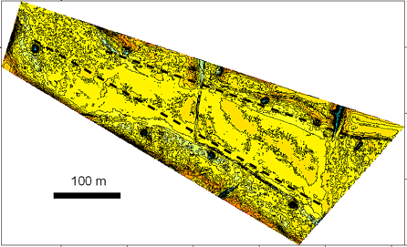
Fig. 15. Palling Lampertsham suggested crater chains.
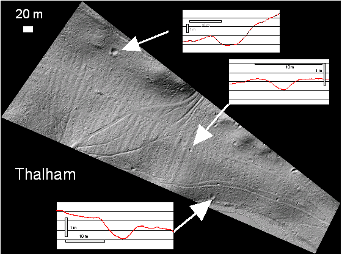
Fig. 16. The Thalham cluster of estimated 20 – 30 craters with rim wall in the DTM shadowed relief map (see the diametral DTM profiles).
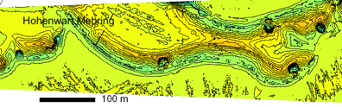
Fig. 17. An irregular cluster of six craters along the terrain ridges. Presumably, other craters in a more regular cluster have been rapidly eroded by post-impact stronger flooding.
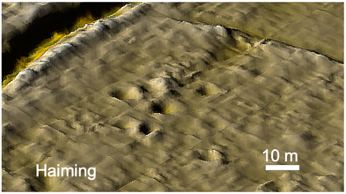
Fig. 18. A cluster of several closely spaced smaller craters is observed in many places in the Chiemgau crater strewn field.
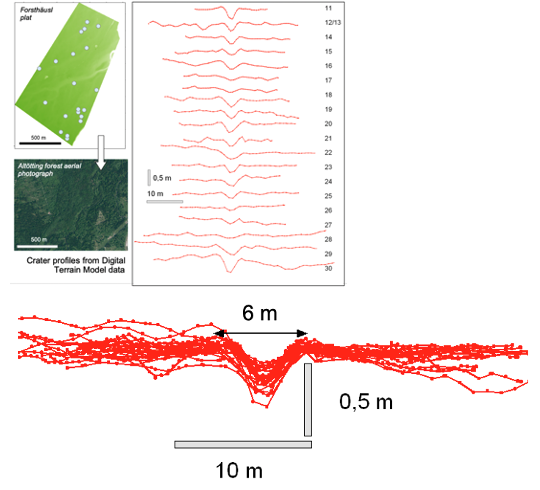
Fig. 19. At least 20 smaller craters with rim walls are distributed in a larger forest patch and are only made visible by high-resolution DTM given the low morphology. The stacking of diametric DTM profiles suggests a fairly uniform cloud of impacting projectiles.
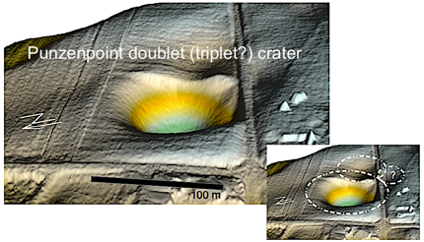
Fig. 20. The Punzenpoint multiple impact crater.
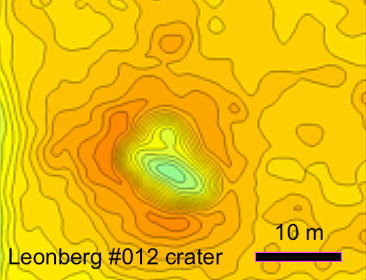
Fig. 21. The Leonberg multiplex impact crater.
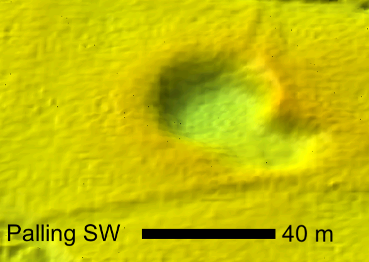
Fig. 22. The Palling SW doublet impact crater.
H: Crater structures, circular in outline, show internal ring features instead of a central morphological depression.
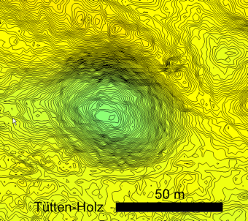
Fig. 23. One of the Tütten-Holz craters (Fig. 14) with stair-like inner ring border.
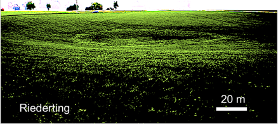
Fig. 24. An inner ring structure of the Riederting crater.
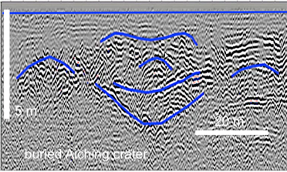
Fig. 25. Ground penetrating radar (GPR) profile reveals a complex inner wall structure of the Aiching semi crater (Fig. 7).
J: occasionally linear ejecta (herringbone features)
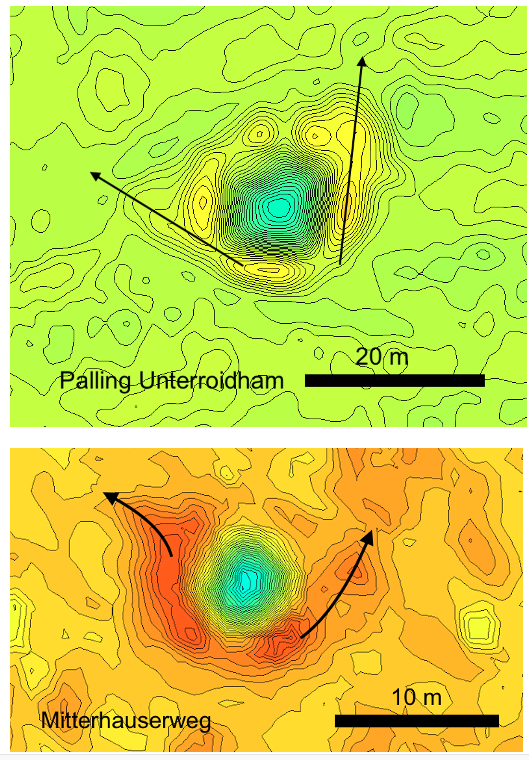
Fig. 26. Wyoming craters with herringbone ejecta formation find their counterparts in the Chiemgau impact strewn field.
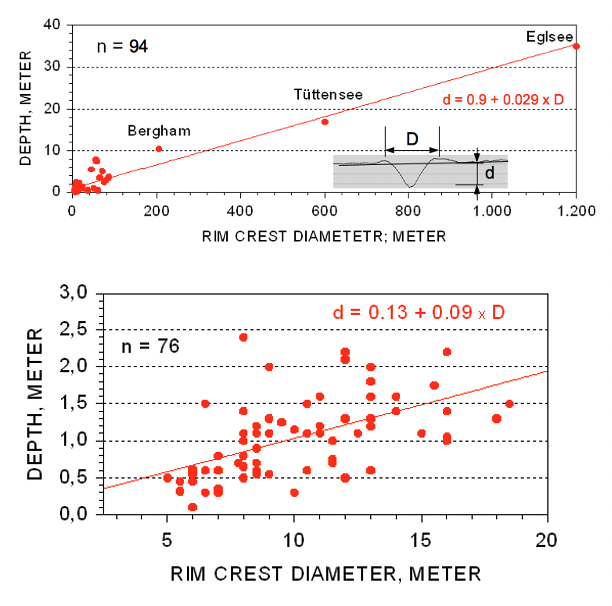
Fig. 27. Depth – diameter dependence for Chiemgau strewn field craters from DTM data with linear regression lines. upper: for all 94 studied craters, lower: for 76 smaller craters. Disregarding the bigger Tüttensee and Eglsee craters, the ratios are not basically different from the Wyoming strewn field.
3.3 Petrographic and mineralogical features – accretionary lapilli, shock metamorphism
Accretionary lapilli Chiemgau impact
Lapilli occur widely and are easily extracted from suitable soils with a stronger magnet. This is usually because they have a core of the strongly magnetic iron silicides xifengite or gupeiite, which are also of great importance in the Chiemgau impact strewn field in many other respects (Rappenglück et al 2017, and references therein).
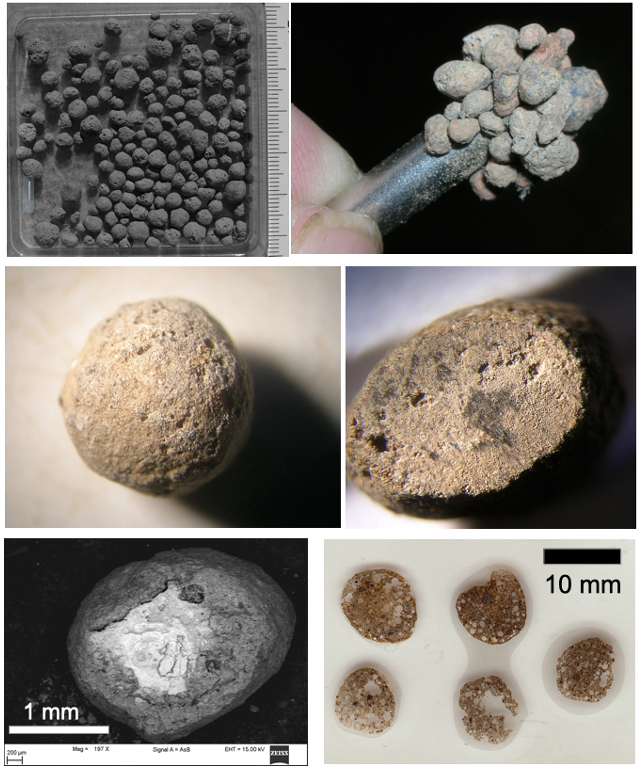
Fig. 28. Accretionary lapilli from the Chiemgau impact strewn field. From upper left to lower right: typical yield from a magnet exploitation. – Magnetic lapilli. – Single lapillo spherule. – Iron silicide core of a lapillo. – SEM image: typical onion skin structure of a Chiemgau impact lapillo. – Thin section of Chiemgau impact lapilli.
Brittle fractures
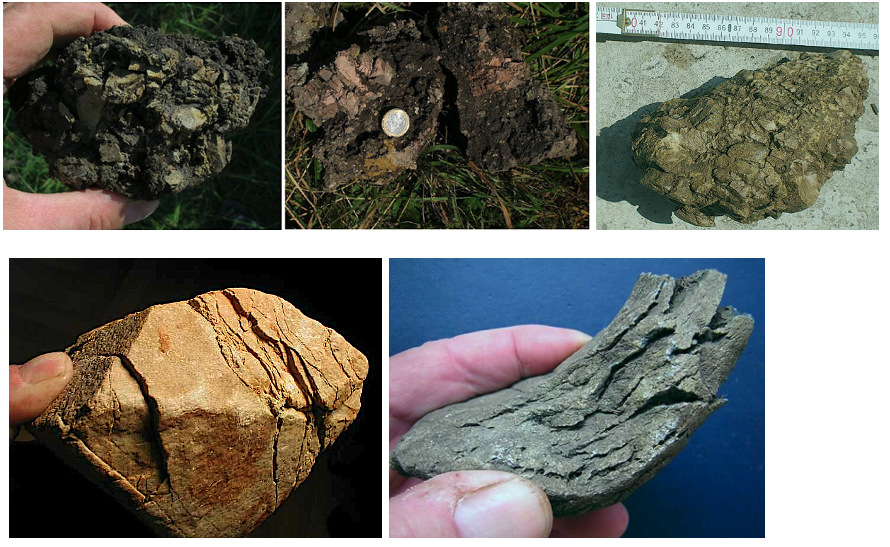
Fig. 29. Brittle fracturing, macro: Intense cobble brecciation and squeezing under high confining pressure enabling coherence. To this day, critics and deniers of the Chiemgau impact never tire of attributing these extreme deformations of the Quaternary cobbles and boulders to tectonic stress in the Alps. How these coherently crushed and squeezed boulders should have survived a transport from the mountains in torrential glacial rivers over tens of kilometers remains unanswered.
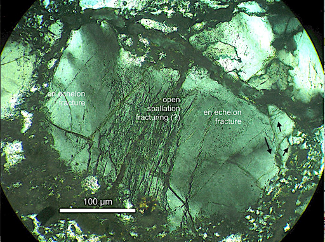
Fig. 30. Quartz grain, brittle fracture, micro. Photomicrograph, crossed polarizers. – One example only out of a volume of comparable mineral deformations in thin sections from the Chiemgau impact strewn field:
Shock metamorphism Chiemgau impact
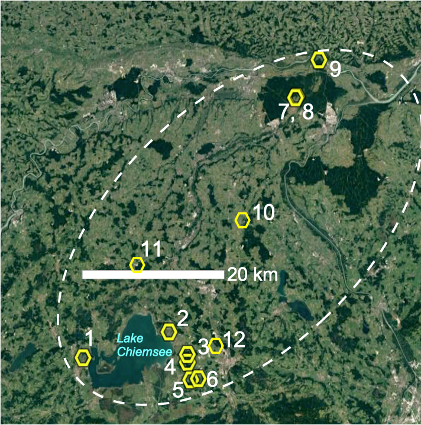
Fig. 31. Location map for shock effects (shock metamorphism) in the Chiemgau impact strewn field. 1 = Mauerkirchen, 2 = Stöttham, 3 = Innerlohen, 4 = Kaltenbach, 5 = Tüttensee, 6 = Mühlbach, 7 = Emmerting #004, 8 = Emmerting #005, 9 = Schatzgrube #001, 10 = Kirchweidach, 11 = Vogelöd, 12 = Riederting.
Planar deformation features (PDF)
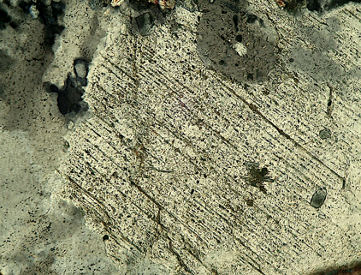
Fig. 32. Quartz grain with decorated PDF. A primary set is crossed by a few fainter sets better to be identified under the microscope.
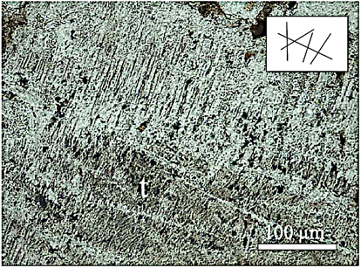
Fig. 33. Quartz grain with multiple sets of PDF (five at least). Several sets are only seen on rotating the microscope stage. t = spot of toasted quartz.
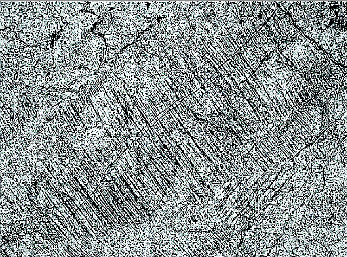
Fig. 34. Quartz grain: two sets of distinct PDF crossed by multiple sets of fainter PDF.
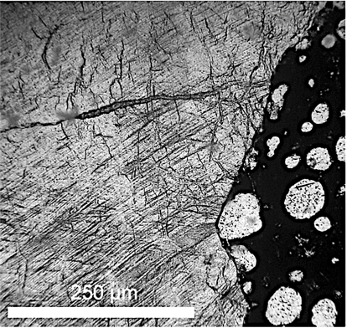
Fig. 35. Quartz with planar deformation features (PDFs) and sets of irregular discontinuous subparallel fractures in contact with dark vesicular glass. The slightly bent PDFs reflect a slightly deformed crystal lattice. Photomicrograph, plane parallel light, field width 480 μm.
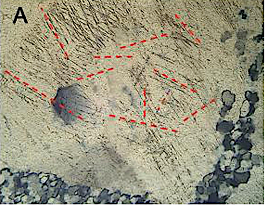
Fig. 36. Photomicrograph of multiple (11 at least as indicated) sets of PDFs in a quartz grain, quartzite. Crossed polarizers; field width 1100 µm. Crossing PDF in unusual signature.
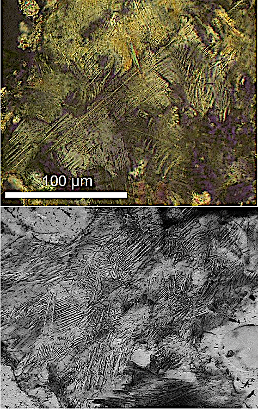
Fig. 37. Multiple sets of PDF in feldspar (plagioclase). Thin section, photomicrograph. Detail below. Typical the ladder structure of crossing PDF in shocked feldspar.
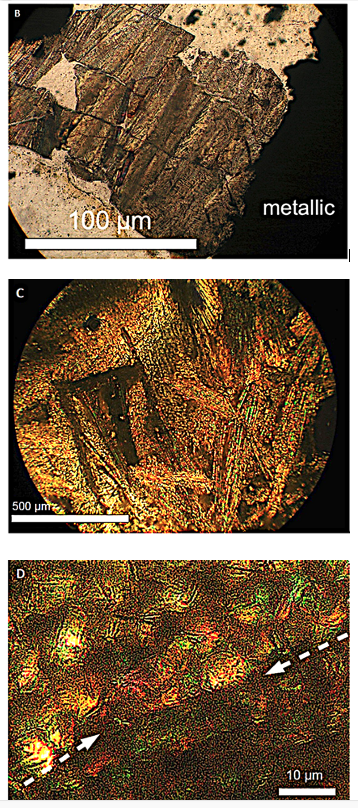
Fig. 38. Various aspects of PDF and mosaicism in amphibole/hornblende minerals in strongly shocked amphibolite.
Diaplectic glass in shocked Chiemgau impact rocks
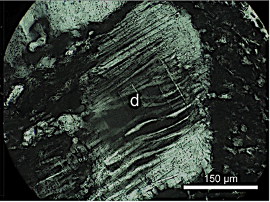
Fig. 39. Sets of PDFs in quartz passing into diaplectic glass (d). Crossed polarizers.
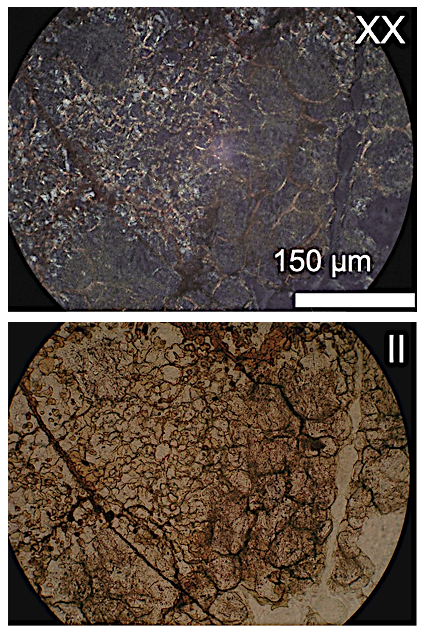
Fig. 40 Ballen structures in diaplectic silica glass.
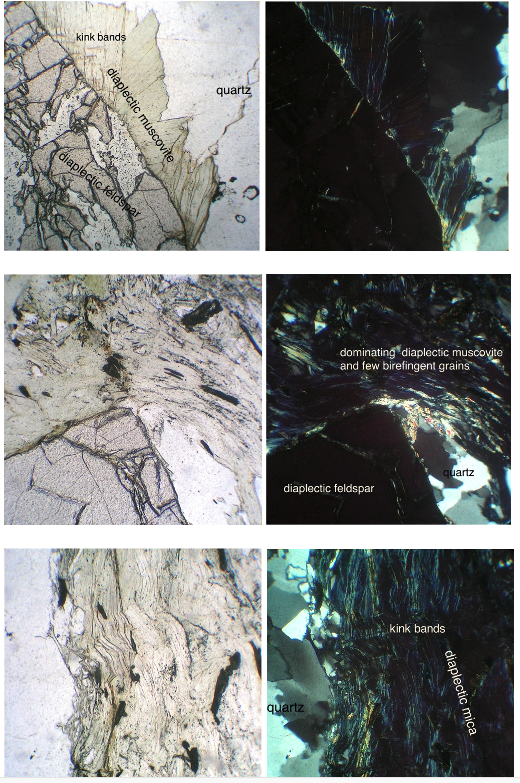
Fig. 41. Diaplectic glass from feldspar and muscovite in the #001 Schatzgrube crater (Chiemgau impact), established as a Carancas, Peru, “twin” crater; see Fig. 58 and Ernstson (2012). Diaplectic mica is a new impact shock modification reported for the first time in the Chiemgau impact event (Ernstson 2012).
Extreme kink banding in mica (tectonics excluded)
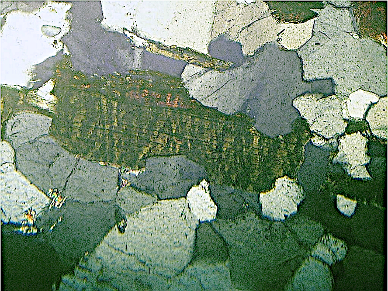
Fig. 42. Two sets of dense kink banding in biotite; gneiss. Crossed polarizers, field width 1 mm.
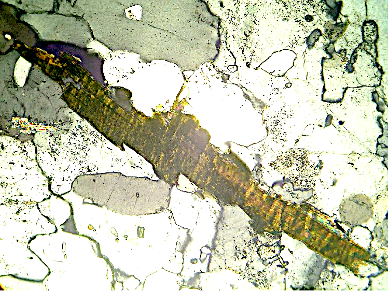
Fig. 43. Dense kink banding of slightly varying orientation in biotite; gneiss. The kink-band width is only 10 – 20 µm on average. Crossed polarizers, field width 1.4 mm.
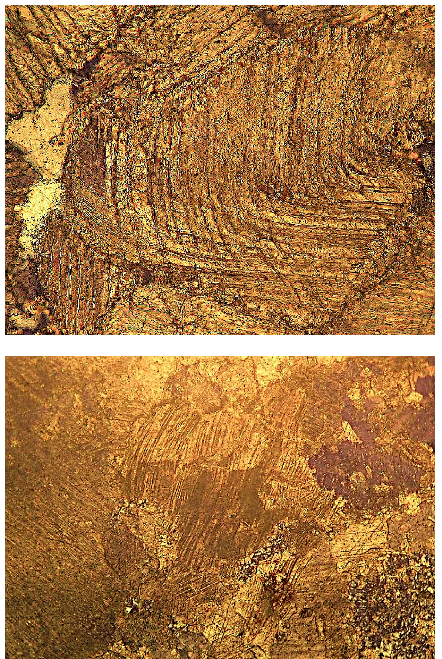
Fig. 44. Strongly deformed calcite exhibiting multiple sets of plastically deformed micro twins. Calcite dikelet in quartzite. Crossed polarizers, field widths 480 µm. The spacing of the micro twins is often only 2 µm.
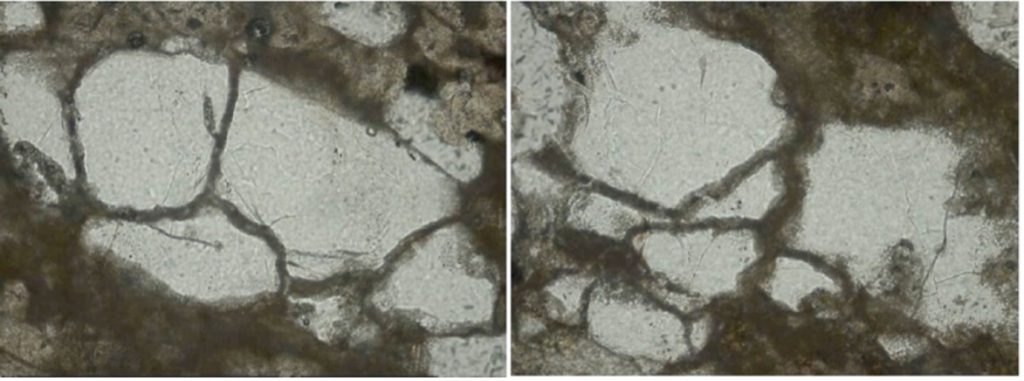
Fig. 45. Shocked quartz grains with open tensile spallation fractures filled with recrystallized impact melt glass. Note that for geometrical reasons the open tensile fractures largely mirror the outer grain surface due to the spallation reflection at the free surface. Field widths 0.8 mm.
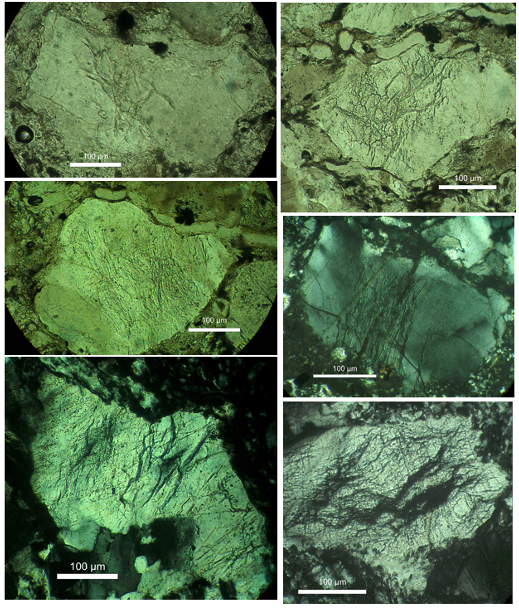
Fig. 46. Quartz grains with multiple subparallel fracturing remarkably focused to the grain interior. This can be explained best by impact shock spallation and interference of tensile rarefaction waves reflected from the free surfaces of the grain boundaries. In some parts the quartz appears to have been transformed to diaplectic glass. Crossed polarizers.
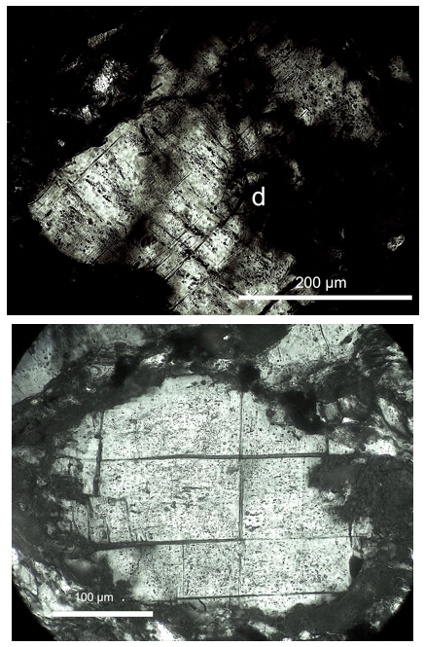
Fig. 47. Shock spallation: open planar tensile fractures in grains of pseudo-cubic quartz. Spots of diaplectic glass (d) in the upper grain.
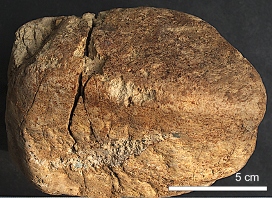
Fig. 48. Macroscopic impact shock spallation abundantly observed in the Chiemgau impact strewn field. Despite the widely open, only piecewise tensile fracture the quartzite cobble has remained coherent, which is also the case in the following examples.
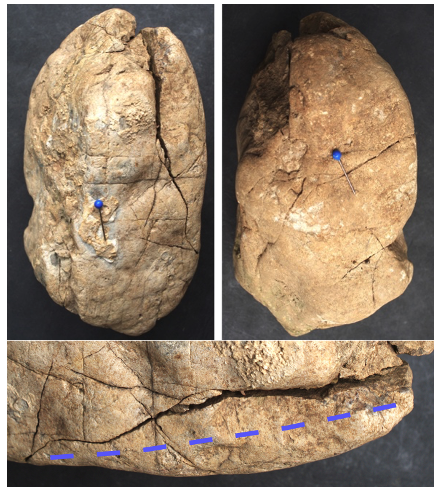
Fig. 49. Like in Fig. 48: fracture-mechanically peculiar shock spallation effect. Note again that the open tensile fracture mirrors the cobble’s free surface. Front and rear.
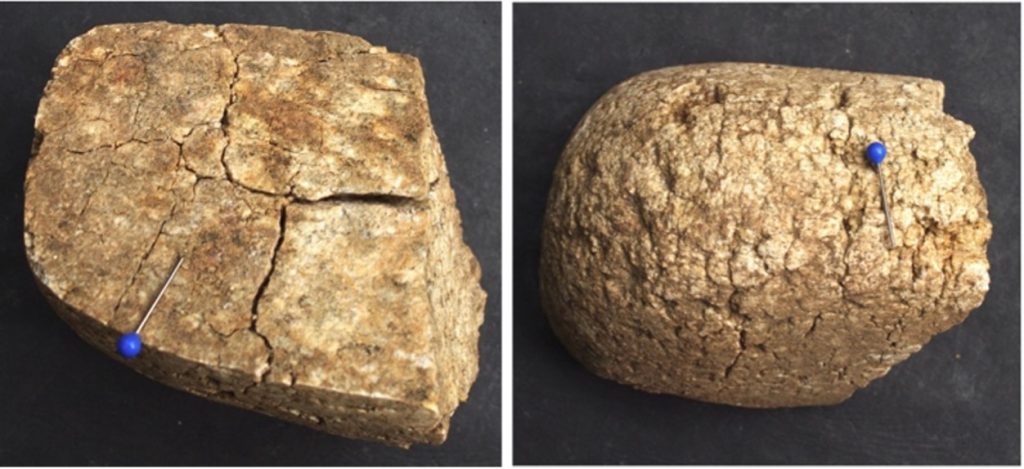
Fig. 50. And one more example.
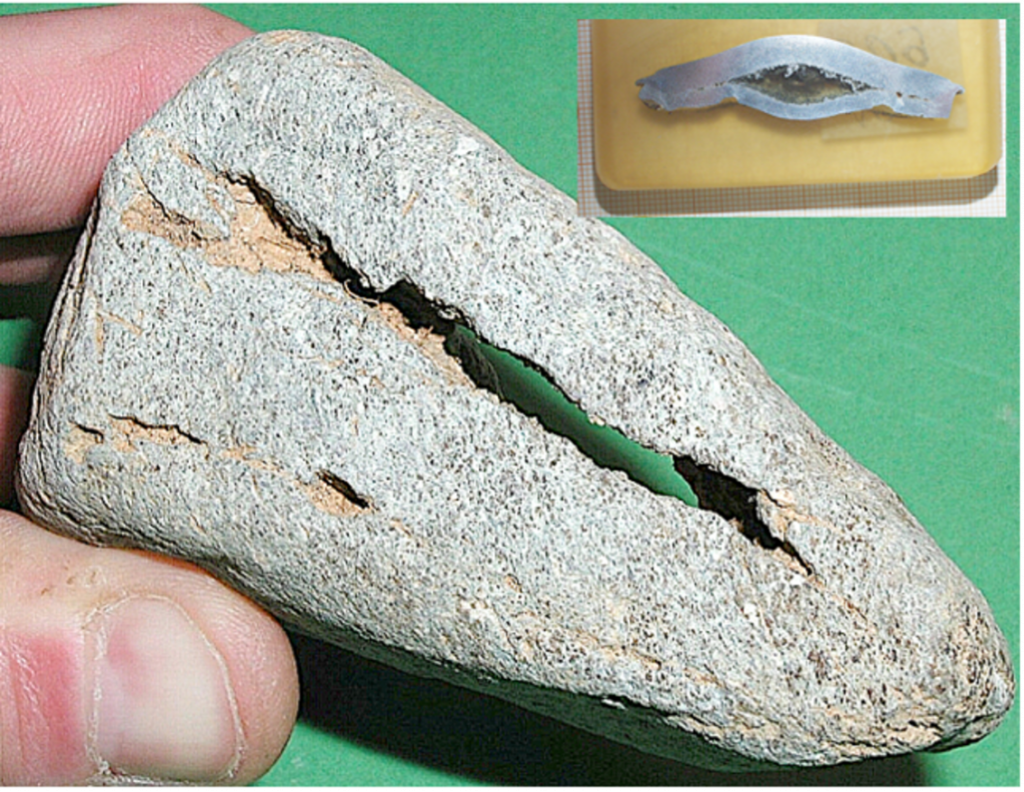
Fig. 51. Impact shock spallation in nature and experiment. Chiemgau impact open rock spallation fracture and experimentally produced analogue shock spallation in ARMCO iron (M. Hiltl).
A shatter cone as impact proof in the Chiemgau impact strewn field
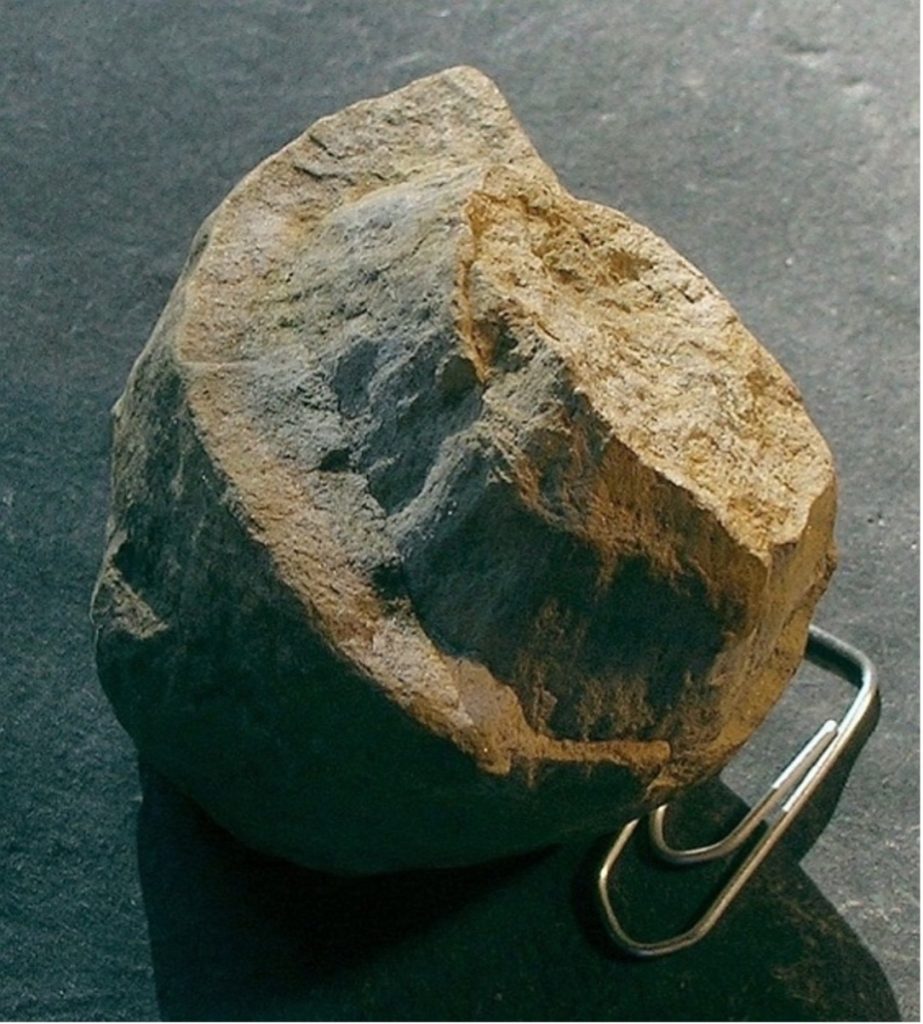
Fig. 52. A Chiemgau shatter cone sample with counter-oriented cones not rare in other impact structures.
4 Gravimetry and the search for a primary crater.
In their article and identification of a primary crater, Kenkmann et al. study gravity maps of regions that might be candidates for location, which in principle would be a reasonable approach. But here Kenkmann et al. make a serious mistake. In the direction of their four intersecting directional beams (their Fig. 1), they detect a negative gravity free-air anomaly with a NW-SE extent of about 130 km and a -65 mGal anomaly that is supposed to split into two roughly circular negative portions, each about 65 km in diameter. Here, wishful thinking seems to determine the possible assignment to the crater field. The free-air anomaly, which considers only the altitude dependence of the measuring station, is completely out of place. Instead, the Bouguer anomaly with the additional correction of the Bouguer plate should be calculated and presented here.
This is exactly what we have taken from the published literature in Figs. 53 and 54. The illustrated Bouguer maps, which would also have been available easily to Kenkmann et al., show a completely irregular pattern of Bouguer anomalies with amplitudes around roughly -150 mGal. There is not the slightest indication for a location of a 50 km impact structure there.
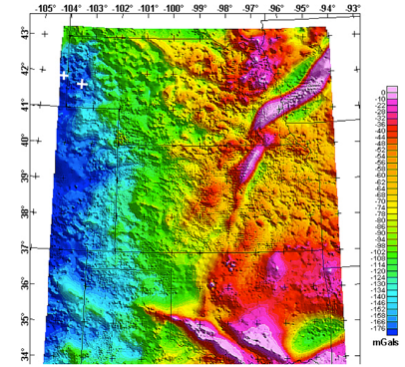
Fig. 53. Bouguer gravity map of Nebraska and adjacent states. The crosses mark the locations of the free-air anomalies of a suspected primary crater (see text). https://pubs.usgs.gov/ds/2005/138/nko_boug.html.
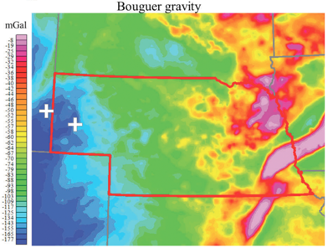
Fig. 54. Bouguer gravity map of Nebraska in more detail. The crosses mark the locations of the free-air anomalies of a suspected primary crater (see text).
At most, the isolated negative gravity anomaly northwest of Douglas in the Bouguer gravity map of Wyoming (Fig. 55) could be part of a larger multiple primary impact, which could be investigated further (e.g., identifying a larger regional field with the calculation and delineation of a residual field that might be more suggestive of an impact structure. The present structure does not look very promising.
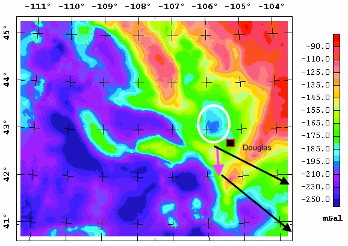
Fig. 55. Bouguer gravity map of Wyoming showing a negative anomaly with a central anomaly of relative -40 – -30 mGal (ellipse) northwest of Douglas and north of the proposed secondary crater field (pink arrow). The black arrows mark the corridor of suggested trajectories for proposed secondary crater projectiles (see text). https://pubs.usgs.gov/of/2000/ofr-00-0198/html/wy_boug.htm
5 The Wyoming craters and the axes asymmetry – Kenkmann et al. Supplemental Material
A major argument for the origin of the Wyoming crater strewn field as ejecta impacts from a large primary crater is the elongation of portions of the craters in a limited direction from which the associated projectiles came and produced the elliptical to ovoid structures on oblique impact. We have taken from the Supplemental material in the Kenkmann et al. article (file:///Users/kordernstson/Downloads/B36196_SuppMat.pdf) the original measured data for the 31 craters designated and measured as proven, and analyzed them in detail. Fig. 56. shows that the result with the construction of the four acute-angled, intersecting corridors in Fig. 1 of the Kenkmann et al. article obviously originates from a wishful thinking and the listed numbers cannot verify this also in rudiments. To this end, we state: Of the total of 31 craters measured, 15 alone have an eccentricity e = 1, that is, they are circular. With four more with e ≤ 1.2 (1.12, 1.13, 1.17, 1.05, thus practically also round) 19 of the 31 measured craters are simply round, thus significantly more than half. As if that were not enough, according to our Fig. 56 and separated by clusters, of the 23 measurements in cluster SM (corridor B) have an eccentricity e = 1 and 14 craters have an e ≤ 1.2, so with the latter, again more than half are considered round. It becomes more perplexing with corridor A (cluster WR). Here there are only two measured craters with identical e = 1.24, but with exactly the same strike directions for both axes. It remains puzzling how a single axis can span a triangle. The same is noted for cluster PCR (corridor D), where a single surveyed crater, which is also circular at e = 1.05, can span the triangular corridor.
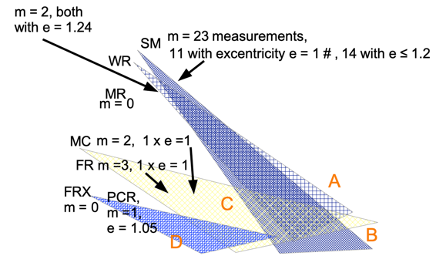
Fig. 56. Traced after Kenkmann et al. (2022, Fig. 1) using their Supplemental Material data. Adopted are the courses of the four corridors (down cut off in the southeast) spanned by the surveyed axes of the elongated craters in each cluster, whose intersection with the free-air anomaly (Fig. 1 in the Kenkmann et al. paper) should define the presumed location of a primary crater. Statistically, this construction has zero value for the intended purpose (see text).
6 Discussion
At the end of our comparison of the postulated Wyoming secondary crater field (Kenkmann et al. 2022) with the Chiemgau impact crater strewn field, the discussion will highlight several key points that must mark the secondary crater field as probably pure fiction. It can be assumed that the undoubtedly existing strewn field is nothing else than the result of a primary impact of a previously already disintegrated asteroid or comet.
A secondary crater field, as is well known from extraterrestrial impacts, logically requires a primary large impact crater. Kenkmann et al. are not able to show such a crater. Such a primary crater does not exist so far. The negative gravimetric free-air anomaly (which is not shown in the article) is a fundamental methodological mistake, because geophysically relevant is the Bouguer anomaly. At the location of the free-air anomalies described by Kenkmann et al. the map of the Bouguer anomalies (our figures 53 and 54) does not show any special feature, which does not even suggest a large impact structure there. It is not clear to us why the Bouguer map, which can be easily downloaded from the Internet, was ignored, as it is used and presented as a matter of course in impact structures in worldwide impact research.
The elliptical to ovoid elongation of the postulated secondary craters (but also not of all) as assumed “signpost” to the primary crater remains without significance, because such an asymmetry can arise in both cases: at the impact of the single projectiles of a previously disintegrated asteroid or comet, or at the impact of the ejecta launched from a primary crater (Fig. 57). In many cases it appears that apparently elongated craters, especially when they are no longer fresh as in Wyoming, are simply the result of overlapping double craters, as is very well observed in the DTM for many craters in the Chiemgau strewn field. An apparent ellipticity of a crater then also results in only an apparent impact direction.
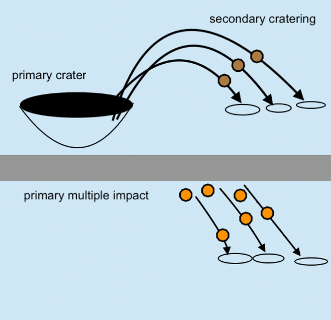
Fig. 57. Both secondary impact cratering and primary multiple impacts can lead to strewn fields with elongated craters.
Here is in addition still the substantial finding to state that more than half of the 31 measured Wyoming craters are mixed up practically circular. The estimation suggests itself that such a mixture of round and elliptical craters could take place rather with a primary impact with an asteroid or comet disintegrated before into individual parts, where internal explosions and collisions could easily influence the further trajectories.
A serious point missed in the Kenkmann et al. article is that while the entire survey procedures are described in detail, for not a single one of the craters is the topography of a digital terrain model with contour lines shown, against which the reader can trace the determination of the eccentricities and strike directions. Instead, one sees by vegetation washed out aerial photographs with the drawn axes (Supplemental Materials), which one could lay with irregularly formed craters also quite differently.
Hence, we supplement the remarks of the previous chapter, in which we show that the entire construction of the assumed entry corridors according to the listed data in the Supplemental Material of Kenkmann et al. is also absolutely meaningless to manipulative. Here and in connection with the wrong gravimetry it has to be pointed out that the possible location of the primary impact presented in Fig. 1 of Kenkmann et al. is pure fiction and not supported by anything.
At this point we question why it is noted rather casually by Kenkmann et al. that no extraneous material was found in the postulated secondary craters that could have originated as landed ejecta from a primary crater. This is to be explained, so the authors, that lithofacially no difference should exist between the target of the primary crater (which – we remind – has not appeared anywhere yet) and the targets of the secondary craters.
Another argument by Kenkmann et al. goes to the heart of our comment article. In their discussion of other impact scenarios, they claim that the Wyoming strewn field, at 90 km long, is far too large to have been produced by a direct impact of a multiple projectile. A twenty years old theoretical modeling is cited as justification (Artemieva and Shuvalov 2001), which is said to prove that breakup during atmospheric traverse into fragments spaced more than 1 km apart is not possible.
Here, Kenkmann et al. list the terrestrial crater scattering fields for comparison, which we adopt as a citation from the article: “Morasko, Odessa, Wabar, Henbury, Sikhote Alin, Kaalijärv, and Macha, which are all densely clustered”.
Apart from us, all meteorite enthusiasts and meteorite researchers should rub their eyes in astonishment, why of all things the long known strewn field of Campo del Cielo in Argentina does not appear in the compilation. With an elliptical extension of nearly 20 km length and about 3 km width and 26 craters it exceeds the fields listed by Kenkmann et al. by a considerable amount. Of the 26 structures, at least 4 are listed as explosion craters (Wright et al. 2007) (the others as penetration craters), lined up in three each 3 km apart.
Also in Argentina is the large impact crater strewn field of Bajada del Diablo, which has been studied for a good decade (Acevedo et al. 2009, 2012, Prezzi et al. 2010, 2016, Orgeira et al. 2017). More than 80 (according to Acevedo et al. (2012) ca 180) circular or elliptical (Wyoming!) craters in a scattering ellipse with four separate clusters of craters (Acevedo 2012), some with ring walls, are observed. Detailed morphological analyses and geophysical measurements (geomagnetics, electromagnetics, gravimetry) (Prezzi et al. 2016), as well as evidence of breccias and glasses (Acevedo 2012) and an unusual accumulation of extraterrestrial spherules (Orgeira et al. 2017), strongly support the impact hypothesis. Early objections emphasized the basaltic target, with objections from regional geologists still forgetting the purely statistical distribution of impacts in principle on any subsurface. Formation continues to be the subject of study and debate, with the lack of evidence of clear shock effects highlighted by critics to date. For the origin of the strewn field, Acevedo et al. (2012) discuss the impact of a very low-density asteroid of the rubble pile type or a comet. They prefer the latter because of the lack of meteorites so far, which could point in the direction of the Wyoming strewn field.
The Chiemgau impact strewn field implication
Why do we write here in detail about the highly interesting Bajada del Diablo probable large impact crater strewn field? It is not mentioned by Kenkmann et al. in their article with any word.
This leads to the main point of our commentary article with the comparison of the Wyoming crater strewn field with the Chiemgau impact crater strewn field, which, as already noted in the introduction, is mentioned by Kenkmann et al. despite extensive literature presence with not a single word.
Therefore, in this discussion, we once again compile the most important features, which show that, figuratively speaking, both strewn fields with their findings can be superimposed almost exactly, so to speak.
— Extension of the scatter fields (Fig. 2); roughly elliptical elongation.
— Number of structures: 90 (proven and suspected; Wyoming) and more than 100 (suspected more than 200; Chiemgau)
— Crater morphology and structures:
– Crater diameter: 10 m – 70 m (Wyoming); a few meters to a few 100 m (Chiemgau; 1 larger crater 1.3 km in diameter).
– Ratio of crater depth to crater diameter d/D inaccurate 0.1 to 0.3 (Wyoming); 0.1 – 0.04 (Chiemgau, Fig. 27).
– Shape of craters, equally Wyoming (fresh craters) and Chiemgau: circular (Fig. 4), elliptical to ovoid (Fig. 5), irregular (Fig.6)
– Both circular and elongated craters intermixed
– steep crater walls and ring walls (Fig. 7).
– Overturned ejecta flaps (Fig. 8 and 9)
– Proximal ejecta blankets (Figs. 10 – 13)
– Irregular crater clusters, crater chains in which craters partially overlap (Figs. 14 – 22)
– Craters with internal ring structures (Figs. 23 – 25)
– linear ejecta “herringbone features” (Fig. 26)
— Accretionary lapilli (Fig. 28)
— Brittle fractures (Fig. 29 and Fig. 30)
— Shock effects, shock metamorphism Figs. 31 – 51)
— Shatter cones (Chiemgau only, Fig. 52)
The Impossible Fact (poem, Christian Morgenstern): And he comes to the conclusion: … that which must not, can not be.
On September 15, 2007, a stone meteorite about half a meter in size struck near Carancas, a small town on the border with Peru, creating an impact crater about 13 meters in diameter.
The Carancas crater featured something totally unexpected because according to the till then established “laws of impact” such a crater created by a hypervelocity impact of an estimated 0.5-1 m stony meteorite seemed completely impossible (see e.g., Reimold 2006, 2007). And consequently Schultz et al. (2008) in their LPSC abstract article on the Carancas impact are beginning their text with the nice statement: “The Carancas impact crater (just before noon on September 15, 2007) should not have happened.”
We need not especially emphasize that this statement concerns the arguments earlier formulated with unshakeable conviction that the Chiemgau impact with lots of small craters cannot (“must not?”, Morgenstern) exist (Reimold 2006, 2007, Kenkmann 2007, Wünnemann et al. 2007).
We assume that the quoted TV program with the appearance of Reimold and Kenkmann was filmed before the Carancas impact and therefore the common claim of the Berlin impact researchers that the Chiemgau impact could not exist because of the smallness of the craters and the big size of the scattering ellipse was taken ad absurdum. This is especially underlined by the fact that the Carancas crater practically has a twin crater in the Chiemgau strewn field (Fig. 58) with remarkably strong shock effects (Fig. 41) (from Ernstson 2017).
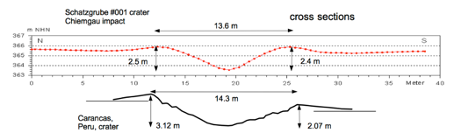
Fig. 58. Cross section of the Schatzgrube #001 crater (Fig. 4, Fig. 8) in comparison with the nearly identical cross section of the Carancas meteorite crater. Carancas section and data redrawn from Kenkmann et al. 2009).
With this pretty comparison we end here the discussion with the quotation from the work of Kenkmann et al. to the alleged Wyoming secondary craters, which could not originate from a primary impact, and the reader may make his/her own consideration about it.
Kenkmann et al.: “We therefore suggest. The disruption during atmospheric traverse does not allow a separation of fragments perpendicular to the trajectory by more than 1 km (Artemieva and Shuvalov, 2001). Moreover, all other crater strewn fields are associated with iron meteorites. The scenario of a break-up of a single meteoroid is incompatible with the current size of the crater field and the other new findings and must therefore be rejected. Likewise, multiple airbursts of a single meteoroid at a very high altitude could not explain the wide distribution of small craters. A paired meteoroid, a meteoroid shower, or a tidal break-up of an asteroid prior to atmospheric entry could explain the wider extent of the crater field but not the converging trajectories and the lack of meteorites.”
7 Conclusions
The conclusions can be very brief. The Wyoming secondary crater field is a fiction and not supported by anything. Nowhere is there any robust evidence for the existence of an associated primary crater. Rather the impression suggests itself strongly that the authors had the desire to gain scientific merits with the spectacular proof of the first terrestrial secondary crater field. Instead, the article conveys a scientifically exceedingly poor style with the absolute misappropriation of extensively published literature that could stand in the way of their rash postulate, which raises the question of how such an article could pass a serious peer-review process at the journal. We suggest to the authors from the University of Freiburg to visit, study and learn about the really spectacular crater strewn field of the Chiemgau impact before further investigations in the Wyoming strewn field. It is a bit closer than Wyoming, so to say on the doorstep of the Freiburg authors, only 350 km away.
References
Acevedo, R.D., Ponce, J.F., Rocca, M., Rabassa, J., Corbella, H. (2009): Bajada del Diablo impact crater-strewn field: The largest crater field in the Southern Hemisphere. – Geomorphology, 110, 58-67.
R.D. Acevedo, R.D, Rabassa, J., Ponce, J.F., Martínez, O., Orgeira, M.J., Prezzi, C., Corbella, H., González-Guillot, M., Rocca, M., Subías, I., Vásquez, C. (2012): The Bajada del Diablo astrobleme-strewn field, central Patagonia Argentina: extending the exploration to surrounding areas. – Geomorphology, 169, 151-164.
Artemieva, N.A., and Shuvalov, V.V., 2001, Motion of a
fragmented meteoroid through planetary atmosphere:
Journal of Geophysical Research: Planets, v. 106,
p. 3297–3309, https://doi.org/10.1029/2000JE001264.
Bauer, F., Hiltl, M., Rappenglück, M.A., Ernstson, K. (2019): Trigonal and Cubic FE2SI Polymorphs (Hapkeite) in the Eight Kilograms Find of Natural Iron Silicide from Grabenstätt (Chiemgau, Southeast Germany). – 50th Lunar and Planetary Science Conference, Poster, Abstract #1520, LPI Contrib. 2132. https://www.hou.usra.edu/meetings/lpsc2019/pdf/1520.pdf, Posterhttps://www.hou.usra.edu/meetings/lpsc2019/eposter/1520.pdf
Bauer, F. Hiltl, M., Rappenglück, M.A., Neumair, A., K. Ernstson, K. (2013): Fe2Si (Hapkeite) from the subsoil in the alpine foreland (Southeast Germany): is it associated with an impact? – 76th Annual Meteoritical Society Meeting, Meteoritics & Planetary Science, Volume 48, Issue s1, Abstract #5056.
Bauer, M. Hiltl, M. A. Rappenglück, K. Ernstson (2020): An eight kilogram chunk and more: evidence for a new class of iron silicide meteorites from the Chiemgau impact strewn field (SE Germany). – Modern Problems of Theoretical, Experimental, and Applied Mineralogy (Yushkin Readings – 7-10 December 2020, Syktyvkar, Russia), Proceedings, 359-360. https://verein.chiemgau-impakt.de/wp-content/uploads/2020/07/Papers-2020-Yushkin-Readings.pdf
Ernstson, K. (2012): Chiemgau impact: a probable doublet meteorite crater in Lake Chiemsee. – https://www.impact-structures.com/2012/06/chiemgau-impact-a-probable-doublet-meteorite-crater-in-lake-chiemsee/
Ernstson, K. (2016): Evidence of a meteorite impact-induced tsunami in lake Chiemsee (Southeast Germany) strengthened. – 47th Lunar and Planetary Science Conference, 1263.pdf. Abstract https://www.hou.usra.edu/meetings/lpsc2016/pdf/1263.pdf.
Ernstson, K., T. Shumilova, T.G. (2020): Chiemite — a high PT carbon impactite from shock coalification/carbonization of impact target vegetation. – Modern Problems of Theoretical, Experimental, and Applied Mineralogy (Yushkin Readings – 7-10 December 2020, Syktyvkar, Russia), Proceedings, 363-365. https://verein.chiemgau-impakt.de/wp-content/uploads/2020/07/Papers-2020-Yushkin-Readings.pdf
Ernstson, K., Poßekel, J. (2020): Digital terrain model (DTM) topography of small craters in the Holocene Chiemgau (Germany) meteorite impact strewn field. – 11th Planetary Crater Consortium 2020 (LPI Contrib. 2251), Abstract #2019. https://www.chiemgau-impakt.de/wp-content/uploads/2020/06/PCC-2019.pdf
Ernstson, K., Poßekel, J., Rappenglück, M.A. (2020): Near-ground airburst cratering: petrographic and ground penetrating radar (GPR) evidence for a possibly enlarged Chiemgau Impact event (Bavaria, SE-Germany). – 50th Lunar and Planetary Science Conference, Poster, Abstract #1231. https://www.hou.usra.edu/meetings/lpsc2020/pdf/1231.pdf, Poster https://www.hou.usra.edu/meetings/lpsc2020/eposter/1231.pdf
Ernstson, K., Poßekel, J. (2017): Meteorite Impact „Earthquake“ Features (Rock Liquefaction, Surface Wave Deformations, Seismites) from Ground Penetrating Radar (GPR) and Geoelectric Complex Resistivity/Induced Polarization (IP) Measurements, Chiemgau (Alpine Foreland, Southeast Germany). – 2017 Fall Meeting, AGU, New Orleans, 11-15 Dec. Abstract EP53B-1700 https://agu.confex.com/agu/fm17/meetingapp.cgi/Paper/216911, Poster https://www.chiemgau-impakt.de/wp-content/uploads/2017/08/Poster-2017-AGU.pdf
Ernstson, K., Hilt, M., Neumair, A.: Microtektite-Like Glasses from the Northern Calcareous Alps (Southeast Germany): Evidence of a Proximal Impact Ejecta . – 45th Lunar and Planetary Science Conference, held 17-21 March, 2014 at The Woodlands, Texas. LPI Contribution No. 1777, p.1200. – Abstract
Ernstson, K., Müller, W., Neumair, A. (2013): The proposed Nalbach (Saarland, Germany) impact site: is it a companion to the Chiemgau (Southeast Bavaria, Germany) impact strewn field? – 76th Annual Meteoritical Society Meeting, Meteoritics & Planetary Science, Volume 48, Issue s1, Abstract #5058. – Abstract
Ernstson, K., Shumilova, T.G., Isaenko, S.I., Neumair, A., Rappenglück, M.A. (2013): From biomass to glassy carbon and carbynes: evidence of possible meteorite impact shock coalification and carbonization. – In: Modern problems of theoretical, experimental and applied mineralogy (Yushkin Memorial Seminar–2013): Proceedings of mineralogical seminar with international participation. Syktyvkar: IG Komi SC UB RAS, 2013. 546 p. .
Ernstson, K., Neumair, A. (2011), Geoelectric Complex Resistivity Measurements of Soil Liquefaction Features in Quaternary Sediments of the Alpine Foreland, Germany, Abstract NS23A-1555 presented at 2011 Fall Meeting, AGU, San Francisco, Calif., 5-9 Dec.
Ernstson, K., Sideris, C., Liritzis, I., Neumair, A. (2012): The Chiemgau meteorite impact signature of the Stöttham archaeological site (Southeast Germany). – Mediterranean Archaeology and Archaeometry, 12, 249-259.
Ernstson, K., Mayer, W., Neumair, A., Rappenglück, B., Rappenglück, M.A., Sudhaus, D. and Zeller, K.W. (2010): The Chiemgau crater strewn field: evidence of a Holocene large impact in southeast Bavaria, Germany. – Journal of Siberian Federal University, Engineering & Technology, 1 (2010 3) 72-103.
Hoffmann, V., Rösler, W., Patzelt, A., & Raeymaekers, B. (2005). Characterisation of a small crater-like structure in SE Bavaria, Germany. 68th Annual Meteoritical Society Meeting, Gatlinburg, Abstract #5158. Access: https://www.lpi.usra.edu/meetings/ metsoc2005/pdf/download/alpha_g-i.pdf
Hoffmann, V., Tori, M., Funaki, M. (2006). Peculiar magnetic signature of Fe-Silicide phases and dia- mond/fullerene containing carbon spherules. Travaux Géophysiques XXVII – Abstracts of the 10th „Castle Meeting“ – New Trends in Geomagnetism, Paleo, Rock and Environmental Magnetism, 52–53.
Isaenko, S., Shumilova, T.G., Ernstson, K., Shevchuk, S., Neumair, A., Rappenglück M. (2012): Carbynes and DLC in naturally occurring carbon matter from the Alpine Foreland, South-East Germany: Evidence of a probable new impactite. – European Mineralogical Conference, Vol. 1, EMC2012-217, 2012., European Mineralogical Conference 2012. Abstract
Kenkmann, T., Müller, L., Fraser, A., Cook, D., Sundell, K., Rae, A.S.P. (2022): Secondary cratering on Earth: The Wyoming impact crater field. – GSA Bulletin, published online 11 February 2022.
Kenkmann T. (2007) Statement in a TV documentary report (October 10): Der Chiemgau- Impakt, Faszination Wissen, BR.
Kenkmann T., Artemieva N., and Poelchau M. (2008) The Carancas event on September 15, 2007: meteorite fall, impact conditions, and crater characteristics. 39th Lunar and Planetary Science Conference. Abstract #1094.
Liritzis, I., Zacharias, N.. Polymeris, G.S., Kitis, G., Ernstson, K., Sudhaus, D., Neumair, A., Mayer, W., Rappenglück, M.A., Rappenglück, B. (2010): The Chiemgau meteorite impact and tsunami event (Southeast Germany): first OSL dating. – Mediterranean Archaeology and Archaeometry, Vol. 10, No. 4, pp. 17‐33.
Neumair, A., Ernstson, K. (2013): Peculiar Holocene soil layers: evidence of possible distal ejecta deposits in the Chiemgau region, Southeast Germany – 76th Annual Meteoritical Society Meeting, Meteoritics & Planetary Science, Volume 48, Issue s1, Abstract #5057.
Orgeira, M.J., LCastro, L.N.,Goldmann, G.A., Prezzi, C.B., Sileo, E., Vega, D.R., Franzosi, C., Acevedo, R.D., Martínez, O., Rabassa, J. Ponce, J.F. Tófaloa, O.R. (2017): Extraterrestrial microspherules from Bajada del Diablo, Chubut, Argentina. – Geoscience Frontiers, 8, 137-149.
Poßekel, J., Ernstson, K. (2020): Not just a rimmed bowl: Ground penetrating radar (GPR) imagery of small caters in the Holocene Chiemgau (Germany) meteorite impact strewn field. – 11th Planetary Crater Consortium 2020 (LPI Contrib. 2251), Abstract #2014. https://www.chiemgau-impakt.de/wp-content/uploads/2020/06/PCC-2040.pdf
Poßekel, J., Ernstson, K. (2019): Anatomy of Young Meteorite Craters in a Soft Target (Chiemgau Impact Strewn Field, SE Germany) from Ground Penetrating Radar (GPR) Measurements. – 50th Lunar and Planetary Science Conference, Abstract #1204, LPI Contrib. 2132. https://www.hou.usra.edu/meetings/lpsc2019/pdf/1204.pdf, Poster https://www.hou.usra.edu/meetings/lpsc2019/eposter/1204.pdf
Prezzi, C.B., Orgeira, M.J., Acevedo, R., Ponce, F., Martínez, O., Vásquez, C., Corbella, H., González, M., Rabassa, J. (2010): Bajada del Diablo impact crater-strewn field (Argentina): 3-11 ground magnetic and electromagnetic surveys. – Conference Paper in Bollettino di Geofisica Teorica ed Applicata · January 2010, GeoSur 2010, 3-11, 121-124.
Prezzi, C.B.,Orgeira, M.J., Martinez, O., Acevedo, R.D., Ponce, F., Goldmann, G., Magneres, I., Rabassa, R. (2016): Circular structures of Bajada del Diablo (Argentina): geophysical signatures. Geophysical Journal International, 205, 876–899.
Rappenglück, B., Hiltl, M., Ernstson, K. (2020): Artifact-in-impactite: a new kind of impact rock. Evidence from the Chiemgau meteorite impact in southeast Germany. – Modern Problems of Theoretical, Experimental, and Applied Mineralogy (Yushkin Readings – 7-10 December 2020, Syktyvkar, Russia), Proceedings, 365-367. https://verein.chiemgau-impakt.de/wp-content/uploads/2020/07/Papers-2020-Yushkin-Readings.pdf
Rappenglück, B., Hiltl, M., Ernstson, K. (2019): Metallic Artifact Remnants in a Shock-Metamorphosed Impact Breccia: an Extended View of the Archeological Excavation at Stöttham (Chiemgau, SE-Germany) – 50th Lunar and Planetary Science Conference, Poster, Abstract #1334, LPI Contrib. 2132. https://www.hou.usra.edu/meetings/lpsc2019/pdf/1334.pdf, Poster https://www.hou.usra.edu/meetings/lpsc2019/eposter/1334.pdf
Rappenglück, B., Ernstson, K., Liritzis, I., Mayer, W., Neumair, A., Rappenglück, M., Sudhaus, D. (2012): A prehistoric meteorite impact in Southeast Bavaria (Germany): tracing its cultural implications. – 34th International Geological Congress, 5-10 August 2012 – Brisbane, Australia. Abstract
Rappenglück, B., Hiltl, M., Ernstson, K. (2020c): The Chiemgau Impact: evidence of a Latest Bronze Age/Early Iron Age meteorite impact in the archaeological record, and resulting critical considerations of catastrophism. – 25th Annual Meeting of the European Association of Archaeologists, Bern, 4-7 September 2019, Oxford: BAR publishing.
Rappenglück, B., Hiltl, M., Rappenglück, M., Ernstson, K. (2020b): The Chiemgau Impact — a meteorite impact in the Bronze/Iron Age and its extraordinary appearance in the archaeological record. In: Wolfschmidt, G. (ed.) Himmelswelten und Kosmovisionen — Imaginationen, Modelle, Weltanschauungen. Nuncius Hamburgensis. Beiträge zur Geschichte der Naturwissenschaften, 51, Hamburg: tredition. (Reviewed and accepted, forthcoming).
Rappenglück, B., Hiltl, M., Ernstson, K. (2020a): Exceptional evidence of a meteorite impact at the archaeological site of Stöttham (Chiemgau, SE-Germany). In: Draxler, Sonja, Lippisch, Max E. (eds.) Harmony and Symmetry. Celestial regularities shaping human cultures. – Proceedings of the 26th Annual Meeting of the European Society of Astronomy in Culture, August 27 – September 2018, Graz, Oxford: BAR publishing.
Rappenglück, B., Hiltl, M., Ernstson, K. (2020c): The Chiemgau Impact: evidence of a Latest Bronze Age/Early Iron Age meteorite impact in the archaeological record, and resulting critical considerations of catastrophism. – 25th Annual Meeting of the European Association of Archaeologists, Bern, 4-7 September 2019, Oxford: BAR publishing
Rappenglück, B., Rappenglück, M.A., Ernstson, K., Mayer, W., Neumair, A. Sudhaus, D., Liritzis, I. (2010): The fall of Phaethon: a Greco-Roman geomyth preserves the memory of a meteorite impact in Bavaria (south-east Germany). – Antiquity, 84, 428-439.
Rappenglück, B., Ernstson, K., Mayer, W., Neumair, A. Rappenglück, M.A., Sudhaus, D., and Zeller, K.W.: The Chiemgau impact: An extraordinary case study for the question of Holocene meteorite impacts and their cultural implications. – In: Belmonte, J. A. (ed.), Proceedings of the International Conference on Archaeoastronomy, SEAC 16th 2008 “Cosmology across Cultures. Impact of the Study of the Universe in Human Thinking”, Granada September 8-12, 2008, A.S.P. Conf. Ser., 2009.
Rappenglück, B., Rappenglück, M.A. (2006): Does the myth of Phaethon reflect an impact? – Revising the fall of Phaethon and considering a possible relation to the Chiemgau Impact.
– Mediterranean Archaeology and Archaeometry, Proceedings of the International Conference on Archaeoastronomy, SEAC 14th 2006, „Ancient watching of cosmic space and observation of astronomical phenomena“, Vol. 6, No. 3 (2006), 101-109.
Rappenglück, M.A. (2022): Natural Iron Silicides: A Systematic Review. – Minerals, 12(2), 188; https://doi.org/10.3390/min12020188.
Rappenglück, M.A., Bauer, F., Ernstson, K., Hiltl, M. (2014): Meteorite impact on a micrometer scale: iron silicide, carbide and CAI minerals from the Chiemgau impact event (Germany). – In: Problems and perspectives of modern mineralogy (Yushkin Memorial Seminar–2014) Proceedings of mineralogical seminar with international participation Syktyvkar, Komi Republic, Russia 19–22 May 2014.
Rappenglück, M.A., Bauer, F. Hiltl, M., Neumair, A., K. Ernstson, K. (2013): Calcium-Aluminium-rich Inclusions (CAIs) in iron silicide matter (Xifengite, Gupeiite, Hapkeite): evidence of a cosmic origin – 76th Annual Meteoritical Society Meeting, Meteoritics & Planetary Science, Volume 48, Issue s1, Abstract #5055.
Rappenglück, M.A, Rappenglück, B., Ernstson, K. (2017): Kosmische Kollision in der Frühgeschichte. Der Chiemgau-Impakt: Die Erforschung eines bayerischen Meteoritenkrater-Streufelds. – Zeitschrift für Anomalistik, Band 17, 235 -260.
English translation: Cosmic collision in prehistory The Chiemgau Impact : research in a Bavarian meteorite crater strewn field ( translated from German ) 2019): https://pdfs.semanticscholar.org/0b62/4ca79c834edc46c86e1fa575c70f726608c8.pdf?_ga=2.133770253.2003692324.1598954865-1676338455.1598954865
Reimold W.U. (2006): Press release. – Naturkundemuseum Berlin, Nov. 21. 2006.
Reimold W.U. (2007) Statement in a TV documentary report (October 10): Der Chiemgau- Impakt, Faszination Wissen, BR.
Rösler, W., Hoffmann, V., Raeymaekers, B., Schryvers, D., & Popp, J. (2005). Carbon spherules with dia- monds in soils. Paneth Kolloquium, Abstract PC2005 #026. Access: http://www.paneth.eu/ PanethKolloquium/Archive_files/PanethKolloquium_2005.pdf.
Schüssler, U., Rappenglück, M. A., Ernstson, K., Mayer, W., Rappenglück, B. (2005). Das Impakt-Krater-Streufeld im Chiemgau. European Journal of Mineralogy, 17(1), 124.
Shumilova, T. G., Isaenko S. I., Makeev B. A., Ernstson K., Neumair A., Rappenglück M. A.: Enigmatic Poorly Structured Carbon Substances from the Alpine Foreland, Southeast Germany: Evidence of a Cosmic Relation. 43nd Lunar and Planetary Science Conference (2012), 1430.pdf.
Shumilova, T.G., Isaenko, S.I., Ulyashev, V.V., Makeev, B.A., Rappenglück, M.A., Veligzhanin, A.A., Ernstson, K. (2018): Enigmatic Glass-Like Carbon from the Alpine Foreland, Southeast Germany: A Natural Carbonization Process. – Acta Geologica Sinica (English Edition), Vol. 92, Issue 6, 2179-2200. https://onlinelibrary.wiley.com/doi/10.1111/1755-6724.13722
Tancredi, G. et al. (2009) A meteorite crater on Earth formed on September 15, 2007: The Carancas hypervelocity impact. – Meteoritics & Planetary Science, 44, Nr 12, 1967–1984.
Wright, S.P., Vesconi, M.A., Spagnuolo, M.G., Cerutti, C., Jacob, R.W., Cassidy, W.A. (2007): Explosion craters and penetration funnels in the Campo del Cielo, Argentina crater field. Lunar and Planetary Science XXXVIII (2007) 2017.pdf.
Wünnemann K., Reimold, W.U. and Kenkmann, T. (2007) Postuliertes Impaktereignis im Chiemgau nicht haltbar. – GMIT, 27, 19-21.
Yang, Z. Q., Verbeeck, J., Schryvers, D., Tarcea, N., Popp, J., & Rösler, W. (2008). TEM and Raman charac- terisation of diamond micro- and nanostructures in carbon spherules from upper soils. Diamond & Related Materials, 17, 937–943.